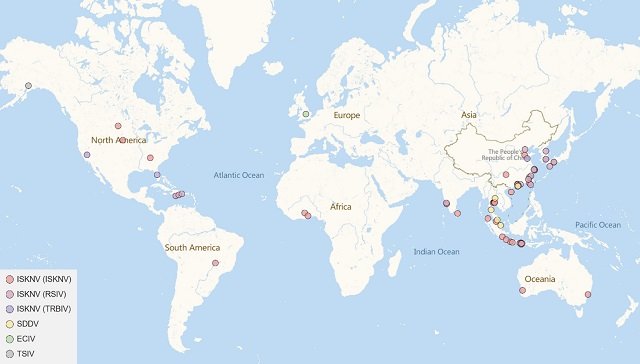
Megalocytivirus, belonging to the Iridoviridae family, has established itself as one of the most concerning viral pathogens for global aquaculture, affecting over 100 species of both freshwater and marine fish, including edible and ornamental fish. This double-stranded DNA virus causes significant economic losses, and its management represents a constant challenge for the aquaculture industry.
A recent scientific review, published in the journal Reviews in Aquaculture by scientists from Sun Yat-Sen University, compiles current knowledge about Megalocytivirus, its epidemiology, pathogenicity, immune evasion, and crucial prevention strategies – vital information for producers, technicians, and researchers in the sector.
What is Megalocytivirus and How is it Classified?
Megalocytiviruses are large viruses (120-200 nm in diameter) with icosahedral symmetry. Currently, two main viral species are primarily recognized within this genus:
- Megalocytivirus pagrus 1: Includes three distinct genotypes:
- Infectious spleen and kidney necrosis virus (ISKNV).
- Red seabream iridovirus (RSIV).
- Turbot reddish body iridovirus (TRBIV). Infection by Megalocytivirus pagrus 1 requires mandatory notification to the World Organisation for Animal Health (WOAH).
- Megalocytivirus lates 1: Comprises a single genotype known as the scale drop disease virus (SDDV).
Recently, other species such as European chub iridovirus (ECIV) and Threespine stickleback iridovirus (TSIV) have been proposed.
Epidemiology: Global Scope and Susceptible Species
Geographic Distribution
Megalocytivirus has a practically worldwide distribution, having been detected on every continent except Antarctica. Asia has been particularly affected, with outbreaks reported from Japan to China, Korea, Thailand, India, and Malaysia, where new strains like SDDV in Asian sea bass (Lates calcarifer) have even been identified. Although considered virus-free, Australia has detected imported cases and remains at risk.
In Europe, although less frequent, it has been found in imported ornamental fish and research fish like zebrafish in Spain, and even native strains like ECIV in England.
North America has seen cases in ornamental and wild fish (USA, Canada, Alaska) and, more recently, significant outbreaks in farmed tilapia and pompano in Central America. In South America, Brazil has reported ISKNV infections in tilapia, while Africa experienced the first devastating ISKNV outbreak in tilapia in Ghana.
Wide Host Range
The ability of Megalocytivirus to infect a vast range of species is notable. It has been documented in over 180 fish species belonging to at least 11 distinct taxonomic orders (Perciformes, Cypriniformes, Siluriformes, Pleuronectiformes, among others).
- ISKNV: Detected in species from at least 7 orders and 22 families. Causes high mortality, especially in fish like the mandarin fish (Siniperca chuatsi) and, recently, in tilapia, the second most farmed fish globally.
- RSIV: Reported in fish from at least 5 orders and 17 families, mainly marine.
- TRBIV: Predominantly affects flatfish (Pleuronectiformes) and Perciformes.
- SDDV: Although more recent, it has been isolated from Asian sea bass (Lates calcarifer), yellowfin seabream (Acanthopagrus latus), and European chub (Squalius cephalus). Fish from the order Perciformes appear to be particularly susceptible.
Transmission Modes
The main route of dissemination is horizontal transmission. Cohabitation studies in the laboratory have shown that the virus can pass between individuals of the same or different species.
The study reports that ISKNV transmission has been confirmed via intraperitoneal injection, muscular injection, immersion, oral route, and cohabitation in mandarin fish. It is crucial to highlight the capacity for transmission between freshwater and marine fish, potentially facilitated by the global trade of ornamental fish, which acts as an important vector for dispersal, especially for ISKNV-type viruses. These viruses have been detected in ornamental fish in over 10 countries, with a risk of spreading to wild species. The virus can also survive in water for up to 48 hours, allowing indirect transmission.
Pathogenicity and Clinical Signs: How Does the Virus Affect Fish?
Megalocytivirus causes severe diseases with high mortality rates, which can exceed 90% in mandarin fish, 40-50% in Asian sea bass, 20-80% in groupers, 60-90% in tilapia, and even 100% in Japanese parrotfish (Oplegnathus fasciatus) or 70% in turbot (Scophthalmus maximus) infected with specific strains.
Typical clinical signs include:
- Anoxia (lack of oxygen), anorexia (loss of appetite).
- Erratic swimming patterns.
- External hemorrhages (head, opercula, jaw, fin base).
- Changes in body color, scale bristling and loss.
- Excess mucus, gill congestion, muscle hemorrhages.
- Swelling and erosion of the spleen and kidney (key affected organs).
- Ascites (fluid accumulation in the abdominal cavity).
Histopathologically, extensive necrosis, vacuolization, and inflammation are observed in internal organs (spleen, kidney, liver, stomach). A characteristic sign is the presence of enlarged (hypertrophied) cells with basophilic inclusions, filled with viral particles, especially in the spleen and kidney. Interestingly, this cell enlargement might be related to the virus’s virulence.
The Crucial Role of the Environment
Environmental factors such as water temperature are determinant in Megalocytivirus outbreaks.
- Warm temperatures favor the disease: For ISKNV in mandarin fish, disease and mortality occur at temperatures >25°C, while at 15-20°C there are no clinical signs. Similarly, TRBIV in turbot causes 60% mortality at 22°C and 100% at 25°C, but not at 20°C or 17°C. RSIV outbreaks typically coincide with temperatures >25°C.
- Persistence at low temperatures: Although fish may not show signs at low temperatures (e.g., 13°C), the virus can be detected, indicating it can persist in the host and cause outbreaks when conditions become favorable.
- Sudden temperature changes: Can stress fish, lowering their immune response and making them more susceptible.
According to the study, it has recently been discovered that low dissolved oxygen levels (hypoxia) (< 2 mg/L) are also a critical factor that can trigger ISKNV outbreaks. Hypoxia activates the hypoxia-inducible factor (HIF)-1 pathway in the fish. The ISKNV virus appears to “hijack” this pathway, using hypoxia response elements in its own genome and viral proteins (like VP077R) to dismantle the inhibitory mechanisms of the HIF pathway, thereby increasing its own replication. This underscores the importance of maintaining optimal dissolved oxygen levels in farms.
Prevention and Control Strategies: Present and Future
Given the complexity of Megalocytivirus, its control requires a multifaceted approach.
- Diagnosis: Early detection is fundamental. Various diagnostic techniques have been developed:
- Traditional methods: Histopathology (observation of enlarged cells), cell culture in sensitive lines, immunofluorescence.
- PCR (Polymerase Chain Reaction): Widely used, including nested PCR for increased sensitivity.
- Recent Advances:
- Multiplex PCR: Allows simultaneous detection and differentiation of various viral genotypes.
- Whole Genome Sequencing (WGS): Using approaches like tiled-PCR, crucial for understanding genomic variation and viral evolution.
- Real-Time PCR (qPCR): TaqMan-based assays offer high sensitivity and specificity for quantifying viral load.
- Digital PCR (ddPCR): Ultra-sensitive, capable of detecting very low viral loads, useful for early diagnosis.
- Isothermal Amplification: Techniques like RPA combined with CRISPR/Cas12a offer rapid, sensitive, and visual detection, ideal for field use.
- Environmental DNA (eDNA): Allows detection of the virus in water before clinical signs appear, promising for proactive surveillance.
- Cross Priming Amplification (CPA): Combined with colorimetric detection, simple and cost-effective. These advancements significantly improve the capacity for rapid and accurate detection, facilitating more effective management strategies.
- Vaccination: The study highlights vaccination as one of the most potent preventive strategies. Considerable progress has been made in developing Megalocytivirus vaccines:
- Inactivated (Killed) Vaccines: Based on inactivated virus (e.g., with formalin). Have demonstrated high efficacy (>90%) and cross-protection between strains (ISKNV and RSIV) in various species (seabream, mandarin fish, spotted seabass, Asian seabass).
- Commercial inactivated vaccines for RSIV (Japan) and ISKNV (China) are already available.
- Disadvantage: Usually require administration by injection, involving individual handling and stress.
- Subunit Vaccines: Utilize specific recombinant viral proteins (e.g., Major Capsid Protein – MCP). Have shown protective efficacy (e.g., 64.3% for ISKNV MCP). Can be enhanced with adjuvants or carriers like nanoparticles.
- Also typically administered by injection.
- DNA Vaccines: Introduce viral genes (e.g., mcp gene or other ORFs) into plasmids. Have been shown to significantly reduce mortality (e.g., 50% for ISKNV orf093).
- Generally by injection, although oral administration with nanoparticles is being investigated.
- Live Attenuated Vaccines (Gene Deletion): Considered very promising, especially for immersion immunization.
- Created by deleting key viral virulence genes (e.g., orf069L, orf022L, orf103r/tk, orf074r).
- These modified viruses have reduced virulence but can still replicate limitedly in the host, inducing strong and lasting immunity.
- Have demonstrated high protection rates (>95%-100%) in mandarin fish, even when administered by immersion.
- Immunization temperature can be key to further attenuate the residual virulence of some strains (e.g., immunizing at 22°C instead of 28°C), making them safer and suitable for industrialized farming models with temperature control.
- Nano-vaccines: Utilize nanomaterials (e.g., carbon nanotubes, chitosan) as carriers or adjuvants to improve delivery and immune response, especially for subunit or DNA vaccines administered by immersion or orally. Have shown promising results in protection (70-89%).
- Viral Vector Vaccines: Utilize other viruses (e.g., baculovirus) modified to express Megalocytivirus proteins. Have shown protection in preliminary studies. It is crucial to develop specific vaccination protocols for each facility and species, and to regularly monitor the effectiveness of the program and the immune status of the fish.
- Inactivated (Killed) Vaccines: Based on inactivated virus (e.g., with formalin). Have demonstrated high efficacy (>90%) and cross-protection between strains (ISKNV and RSIV) in various species (seabream, mandarin fish, spotted seabass, Asian seabass).
- Integrated Management Strategies: Vaccination must be complemented with other strategies:
- Rigorous Biosecurity: Measures to prevent virus entry and spread (quarantine, equipment disinfection, vector control). Follow WOAH guidelines.
- Water Quality Management: Maintain optimal parameters, especially temperature and dissolved oxygen levels.
- Nutrition and Health: Keep fish in optimal nutritional and general health conditions.
- Disease Management:
- Isolation and Treatment: Immediately separate affected fish. A common practice for ISKNV is to cease feeding upon detecting the infection, which seems to reduce mortality, although the mechanism is unclear.
- Disinfection: Thorough cleaning and disinfection of affected facilities with effective agents (e.g., sodium hypochlorite, peracetic acid, benzalkonium, UV, pH changes, heat).
- Specific Pathogen-Free (SPF) Seed Production: This is a vital long-term strategy. It involves selecting virus-free broodstock, maintaining them in controlled and isolated environments, with regular testing and certification. Efforts are already underway to produce SPF mandarin fish seed in China.
- Development of Resistant Lines: Utilize genetic selection or gene editing tools (like CRISPR-Cas9) to develop fish populations more resistant to the disease. Requires ethical and environmental consideration.
Conclusions and Future Perspectives
Megalocytivirus remains a significant challenge for the sustainability of aquaculture. Although much progress has been made in understanding its biology and developing control tools, effective eradication remains elusive.
Future research should focus on:
- Better understanding viral genetic variation and mechanisms of transmission between species.
- Deepening the understanding of environment-virus-host interactions, especially the role of factors like hypoxia.
- Developing more effective and practical oral and immersion vaccines, especially for larvae and small fish.
- Advancing SPF seed production and genetic selection/editing for resistance.
- Cutting-edge technologies such as nanopore sequencing for genomic surveillance, deep learning models to predict host ranges, aquatic organoids to study interactions, and CRISPR-Cas9 to validate key factors and develop therapies will be fundamental.
The combination of advanced research, innovative vaccine development, and the rigorous implementation of integrated management and biosecurity strategies will pave the way for more effective control of diseases caused by Megalocytivirus, ensuring a more sustainable future for aquaculture.
Contact
Jianguo He
School of Marine Sciences, State Key Laboratory for Biocontrol & Southern Marine Science and Engineering Guangdong Laboratory (Zhuhai), Guangdong Province Key Laboratory of Aquatic Economic Animals & Guangdong Provincial Observation and Research Station for Marine Ranching of the Lingdingyang Bay, School of Life Sciences, Sun Yat-Sen University, Guangzhou, People’s Republic of China
Email: lsshjg@mail.sysu.edu.cn
Reference (open access)
Guo, C., He, J., Xu, X., Weng, S., & He, J. (2025). Megalocytivirus: A Review of Epidemiology, Pathogenicity, Immune Evasion, and Prevention Strategies. Reviews in Aquaculture, 17(3), e70025. https://doi.org/10.1111/raq.70025