Environmental DNA (eDNA) is transforming the way scientists study biodiversity, conservation, and ecosystem health. Researchers can monitor species without capturing or directly disturbing them by analyzing DNA traces found in the environment. This technique is revolutionizing ecology by offering a non-invasive and highly efficient method to study wildlife, aquatic ecosystems, and even ancient biodiversity.
eDNA is the fastest-growing biomonitoring tool, driven by two key features: temporal efficiency and sensitivity (Takahashi et al., 2023). But what is the potential of environmental DNA for aquaculture?
eDNA technology has emerged as a pioneering tool in fisheries and aquaculture, providing new approaches for monitoring and managing aquatic ecosystems (Chouhan et al., 2023). In this article, we will explore what environmental DNA is, how it is collected and analyzed, its applications in biodiversity research, and its potential to estimate fish populations in aquaculture ponds or identify potential pathogens.
What is environmental DNA?
Environmental DNA (eDNA) refers to genetic material that organisms release into their surroundings, such as water, soil, air, or even ice. This DNA comes from various sources, including skin cells, mucus, feces, and other biological materials. By collecting and analyzing eDNA, scientists can detect the presence of species without physically capturing them.
Technological advancements in recent years have made eDNA a powerful tool for species detection, ecosystem monitoring, and environmental impact assessments. Unlike traditional sampling methods, which often require capturing individuals, eDNA offers a more ethical and less invasive approach to studying wildlife.
Why es environmental DNA important?
- Non-invasive Monitoring: Unlike traditional studies that may require trapping or tagging animals, eDNA allows scientists to detect species without physical interaction. Takahashi et al. (2023) reported that current eDNA methods are “sufficiently advanced to study fish community composition and replace more invasive traditional methods.”
- High Sensitivity: Even tiny traces of DNA can reveal the presence of elusive or rare species.
- Wide Applications: eDNA is used in biodiversity research, conservation biology, and forensic ecology. Ramey et al., (2024) highlighted eDNA as a useful tool for detecting the presence of Atlantic and Pacific salmon and understanding threats to fish health and habitats.
- Time and Cost Efficiency: Compared to traditional field studies, eDNA analysis is often faster and more cost-effective.
- Biomass Estimation: In aquaculture, eDNA can be used to estimate the number of fish in a pond.
How environmental DNA sampling works
eDNA sampling involves collecting biological material from natural environments, such as water or soil, and analyzing it for genetic markers. Below is a step-by-step overview of the process:
- Sample Collection: Scientists collect environmental samples (e.g., water, sediment, or air) using sterile techniques. For example, Lee et al., (2024) highlighted the effectiveness of plankton nets as a simple and robust eDNA-based tool for detecting viral hemorrhagic septicemia virus (VHSV) in aquaculture water samples.
- Filtration and DNA Extraction: The sample is filtered to concentrate DNA, which is then extracted using laboratory protocols.
- Polymerase Chain Reaction (PCR) or Metabarcoding: Specific DNA sequences are amplified to detect target species.
- Sequencing and Analysis: DNA is sequenced using next-generation sequencing (NGS) or quantitative PCR to identify species present in the sample.
- Data Interpretation: Bioinformatics tools compare DNA sequences with genetic databases to determine the detected organisms.
eDNA technology holds great potential for advancing aquaculture sustainability by enabling precise species monitoring, early disease detection, and improved resource management.
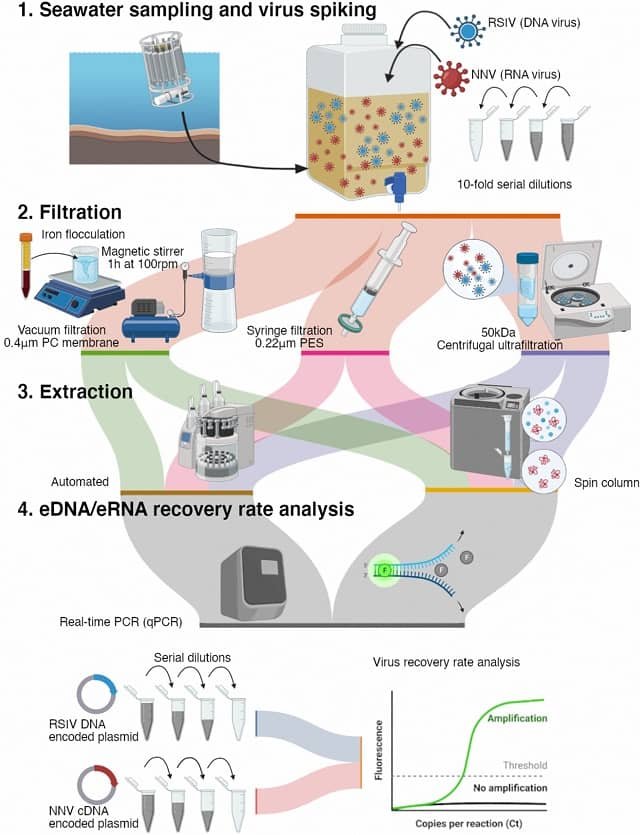
Environmental DNA for biodiversity research and monitoring
eDNA has the potential to revolutionize biodiversity research and monitoring. In this regard, Ge et al., (2025) introduced the Environmental DNA Integrity Index (eDI) to adjust eDNA concentration for estimating carp biomass. The eDI index is calculated as the ratio between long-chain DNA content and short-chain DNA content.
Key applications of eDNA
- Species Inventory and Distribution Mapping: eDNA can be used to identify species present in a particular area, even if they are rare or elusive.
- Early Detection of Invasive Species: eDNA can be used to detect the presence of invasive species at early stages, enabling a rapid response and control measures.
- Monitoring Population Trends: eDNA can be used to track species population trends over time.
- Habitat Quality Assessment: eDNA can be used to evaluate habitat quality and identify areas requiring conservation efforts.
- Detection of Endangered Species: eDNA can be used to detect endangered species, even in remote or inaccessible areas.
Application of environmental DNA in aquaculture
Population estimation
Momin et al. (2024) highlights that eDNA concentration could provide a fast and cost-effective method for measuring biomass and/or abundance, useful for assessing fish populations. In this regard, Benoit et al., (2023) found a positive relationship between environmental DNA (eDNA) concentration and juvenile Chinook salmon biomass. However, the study also emphasizes that using qPCR as an independent method to assess juvenile salmon biomass may only be semi-quantitative with current methods and requires further calibration for field application.
Health monitoring
According to Momin et al. (2024), eDNA monitoring is likely to have a significant impact on the ability of the salmon farming industry and its regulators to detect the presence and abundance of pathogens and other biological threats in the surrounding environment. Krolicka et al., (2022) developed a new qPCR assay specifically for detecting environmental DNA (eDNA) of the salmon parasite Lepeophtheirus salmonis, which is compatible with the Environmental Sample Processor (ESP). This method represents a simple, non-intrusive, and cost-effective alternative for monitoring L. salmonis in the field, providing rapid infection notifications to producers without compromising fish welfare.
Additionally, Chouhan et al. (2023) and Ramey et al. (2024) reported that applying eDNA tools also shows promise for early detection of emerging pathogens or invasive species that could negatively impact fish health, with the potential to promote rapid response and effective mitigation and management strategies.
In this regard, Lo et al., (2023) presented an environmental DNA (eDNA) metabarcoding approach that employs a coastal model with an established database. This approach demonstrated excellent potential for diverse coastal pathogen monitoring and revealed spatiotemporal prevalence mechanisms associated with intensive aquaculture.
However, Aden et al., (2024) warns that implementing viral eDNA/RNA detection tools for pathogen surveillance and disease research in aquaculture farms requires careful consideration of seawater filtration methods.
Environmental impact
Environmental DNA (eDNA) is a powerful tool for detecting interactions between aquaculture ecosystems and species in benthic and pelagic environments (English et al., 2024). In this context, Suzuki et al., (2024) used environmental DNA metabarcoding analysis to determine the environmental impact of Coho salmon (Oncorhynchus kisutch) farming on fish biodiversity in Shizugawa Bay. The study reported that environmental DNA metabarcoding showed that the presence or absence of aquaculture explained approximately 10% of the total variation in fish community composition, a variation comparable to water depth and sampling month.
Similarly, Krkosek et al., (2024), using environmental DNA, determined that pathogens from salmon farms pose a conservation concern for wild salmon in British Columbia (BC). Specifically, three pathogens are prevalent in farmed Atlantic salmon in BC and are transmitted to wild Pacific salmon, being linked to negative impacts on the latter: Piscine orthoreovirus, Tenacibaculum spp., and sea lice (Lepeophtheirus salmonis).
Ecosystem services of aquaculture farms
Bivalve aquaculture farms provide a range of environmental services. Mercaldo-Allen et al., (2021) found that video recording and environmental DNA (eDNA) metabarcoding, applied together, provided a comprehensive approach to describing fish assemblages in structured oyster aquaculture and rocky habitats that are difficult to sample.
Similarly, Li et al., (2024) employed environmental DNA techniques to identify 55 fish species in the mussel farming zone and surrounding natural reefs of Gouqi Island in the East China Sea. Researchers reported that diversity in the mussel farming zone was significantly higher than in the natural reef habitat.
Advantages and limitations of environmental DNA
Advantages of environmental DNA
- Non-invasive: Reduces stress and harm to wildlife.
- Highly sensitive: Detects species at low densities.
- Scalable: Can be used across diverse ecosystems.
- Efficient: Saves time and resources compared to traditional sampling.
Limitations of environmental DNA
- Degradation issues: DNA degrades over time, affecting accuracy.
- Contamination risks: Requires strict laboratory controls.
- Tides and ocean currents can affect eDNA dispersion.
- Presence vs. Abundance: Detects species presence but does not always indicate population size or biometric data (size, age, sex).
- Incomplete databases: Species identification depends on existing genetic reference libraries.
Despite these challenges, advancements in environmental DNA sampling protocols and bioinformatics are improving the reliability of eDNA studies.
Future research and applications of environmental DNA
The future of environmental DNA research looks promising, with innovations expanding its applications. Scientists are developing:
- Real-time environmental DNA detection: Portable devices for on-site genetic analysis. Deeg et al., (2025) developed the EZ-eDNA system, designed around a protective polymer case and using modular components that can be deployed in different configurations with various pump and filter setups.
- Environmental DNA and artificial intelligence: AI-driven analysis for faster and more accurate species identification.
- Genomic reference libraries: Expanding databases for better species matching.
As technology advances, environmental DNA for biodiversity research and monitoring will become even more precise, accessible, and impactful.
Conclusion
Environmental DNA (eDNA) is revolutionizing environmental science by offering a powerful, non-invasive, and highly efficient way to study biodiversity. From tracking endangered species to monitoring ecosystem health, eDNA is shaping the future of conservation and ecology.
As research continues to refine environmental DNA sampling protocols and expand its applications, eDNA will remain at the forefront of environmental monitoring and species conservation. By embracing this technology, scientists, policymakers, and conservationists can work together to protect the rich biodiversity of our planet.
References
Aden Ip, Y. C., Chen, J., Tan, L. Y., Lau, C., Chan, Y. H., Balasubramaniam, R. S., Jovinc Wong, W. Y., Ng, K., Brian Tan, Z. Y., Fernandez, C. J., Chang, S. F., & Yap, H. H. (2024). Establishing environmental DNA and RNA protocols for the simultaneous detection of fish viruses from seawater. Environmental DNA, 6(1), e418. https://doi.org/10.1002/edn3.418
Benoit, N. P., Robinson, K. M., E. Kellogg, C. T., Lemay, M. A., & V. Hunt, B. P. (2023). Using qPCR of environmental DNA (eDNA) to estimate the biomass of juvenile Pacific salmon (Oncorhynchus spp.). Environmental DNA, 5(4), 683-696. https://doi.org/10.1002/edn3.422
Chouhan, N., Dekari, D., Choudhary, B., Singh, A., & Choudhury, T. G. (2023). Environmental DNA (eDNA) technology: Fisheries and aquaculture perspectives. Indian J Anim Health, 62(2), 75-85.
Deeg, C. M., Saunders, R. G., Tam, C., Kaukinen, K., Li, S., Bass, A. L., & Miller, K. M. (2025). EDNA Sampling Systems for Salmon Ecosystem Monitoring. Environmental DNA, 7(1), e70059. https://doi.org/10.1002/edn3.70059
English, G., Lawrence, M. J., McKindsey, C. W., Lacoursière-Roussel, A., Bergeron, H., Gauthier, S., Wringe, B. F., & Trudel, M. (2024). A review of data collection methods used to monitor the associations of wild species with marine aquaculture sites. Reviews in Aquaculture, 16(3), 1160-1185. https://doi.org/10.1111/raq.12890
Ge, Q., Piao, Y., Li, Z., Yang, Y., Pan, M., & Bai, Y. (2025). Environmental DNA integrity index is sensitive for species biomass estimation in freshwater. Science of The Total Environment, 966, 178734. https://doi.org/10.1016/j.scitotenv.2025.178734
Krkosek, M., Bateman, A. W., Bass, A. L., Bugg, W. S., Connors, B. M., Deeg, C. M., Cicco, E. D., Godwin, S., Grimm, J., Krichel, L., Mordecai, G., Morton, A., Peacock, S., Shea, D., Riddell, B., & Miller, K. M. (2024). Pathogens from salmon aquaculture in relation to conservation of wild Pacific salmon in Canada. Science Advances. https://doi.org/adn7118
Krolicka A, Mæland Nilsen M, Klitgaard Hansen B, Wulf Jacobsen M, Provan F, Baussant T (2022) Sea lice (Lepeophtherius salmonis) detection and quantification around aquaculture installations using environmental DNA. PLoS ONE 17(9): e0274736. https://doi.org/10.1371/journal.pone.0274736
Lee, Y., Kang, H., Roh, H., Yun, D., Park, J., Lee, J., Heo, Y., Hong, S. Y., Jang, G., Kwon, M., Han, S., Kim, S., Park, C., & Kim, D. (2024). Enhancing viral detection in aquaculture water through virus-microplankton associations and plankton net concentration. Aquaculture, 590, 741092. https://doi.org/10.1016/j.aquaculture.2024.741092
Li, L., Zhang, S., & Wang, Z. (2024). EDNA technology reveals fish species diversity and ecological corridor function in large raft mussel aquaculture area in the East China Sea. Marine Pollution Bulletin, 209, 117171. https://doi.org/10.1016/j.marpolbul.2024.117171
Lo, L. S. H., Liu, X., Liu, H., Shao, M., Qian, P., & Cheng, J. (2023). Aquaculture bacterial pathogen database: Pathogen monitoring and screening in coastal waters using environmental DNA. Water Research X, 20, 100194. https://doi.org/10.1016/j.wroa.2023.100194
Mercaldo-Allen R, Clark P, Liu Y, Phillips G and others (2021) Exploring video and eDNA metabarcoding methods to assess oyster aquaculture cages as fish habitat. Aquacult Environ Interact 13:277-294. https://doi.org/10.3354/aei00408
Momin, C. M., Baidya, S., Debbarma, S., Yadav, N. K., Chandravashi, S., Debnath, A., … & Lavkush, V. A. (2024). Genetic improvement initiatives in aquaculture. Int J Adv Biochem Res, 8(4S), 441-447.
Ramey, A. M., McKeeman, C. M., Petrou, E. L., Menning, D. M., Russ, O. L., & López, J. A. (2024). Environmental DNA as a Tool for Better Understanding the Distribution, Abundance, And Health of Atlantic Salmon and Pacific Salmon. Fisheries, 49(4), 169-180. https://doi.org/10.1002/fsh.11038
Suzuki, S., Otomo, Y., Dazai, A., Abe, T., & Kondoh, M. (2024). Assessing the impacts of aquaculture on local fish communities using environmental DNA metabarcoding analysis. Environmental DNA, 6(3), e551. https://doi.org/10.1002/edn3.551
Takahashi, M., Saccò, M., Kestel, J. H., Nester, G., Campbell, M. A., Van der Heyde, M., Heydenrych, M. J., Juszkiewicz, D. J., Nevill, P., Dawkins, K. L., Bessey, C., Fernandes, K., Miller, H., Power, M., Mousavi-Derazmahalleh, M., Newton, J. P., White, N. E., Richards, Z. T., & Allentoft, M. E. (2023). Aquatic environmental DNA: A review of the macro-organismal biomonitoring revolution. Science of The Total Environment, 873, 162322. https://doi.org/10.1016/j.scitotenv.2023.162322