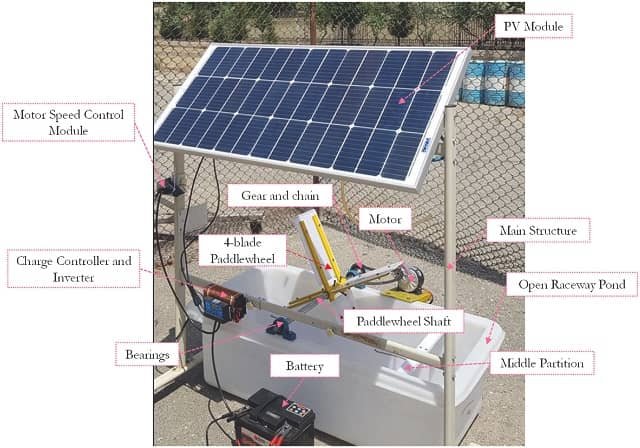
Microalgae culture has garnered significant attention due to its potential applications in various industries, such as biofuels, pharmaceuticals, and nutraceuticals. However, optimizing microalgae production often requires controlled environmental conditions, such as dissolved oxygen (DO) levels, pH, and water temperature.
Scientists from the Fraunhofer Institute for Solar Energy Systems ISE and Tarbiat Modares University (TMU) published a study in the journal Results in Engineering, aiming to develop a small-scale aquavoltaic system and evaluate its impact on these parameters in an open-channel pond, thereby improving microalgae productivity and generating clean energy.
The potential of aquavoltaics
Aquavoltaics offers a promising approach to addressing the challenges faced by the aquaculture industry. Aquavoltaics uses the surface of water bodies to install photovoltaic (PV) panels, allowing for the simultaneous production of electricity and aquatic products. This dual approach can significantly meet the water, food, and energy needs of communities.
By integrating photovoltaic technology into aquaculture, the industry can reduce its reliance on fossil fuels, lower operational costs, and improve sustainability. Key benefits of aquavoltaics include:
- Reduced energy consumption: Photovoltaic panels can generate clean energy to power aquaculture facilities, reducing dependence on fossil fuels and lowering operational costs.
- Improved water quality: The shading provided by photovoltaic panels can help regulate water temperature, reduce evaporation, and improve water quality.
- Increased productivity: The controlled environment created by aquavoltaic systems can optimize conditions for aquatic organism growth, leading to higher productivity.
- Reduced carbon footprint: By generating renewable energy and reducing greenhouse gas emissions, aquavoltaics contributes to climate change mitigation.
Key factors affecting the performance of aquavoltaic systems
Several factors influence the performance of aquavoltaic systems, including:
- Water depth and quality: The depth and quality of the water body can affect the efficiency of photovoltaic panels and the growth of aquatic organisms.
- Design and orientation of photovoltaic panels: The design and orientation of the photovoltaic panels can influence energy production and shading effects.
- Species and aquaculture practices: The type of aquatic organisms being farmed and specific farming practices can affect the overall system performance.
Methodology
The researchers employed a response surface methodology (RSM) with a central composite design (CCD) to investigate the effects of paddlewheel rotation speed, water depth, and response time on DO levels in the channel pond.
The experiments were conducted over a two-week period, varying parameters within predetermined ranges. DO, pH, water temperature, and dead zones were monitored throughout the study.
Results and discussion
The study’s results revealed a significant correlation between paddlewheel rotation speed and water depth in influencing DO levels. Increasing rotation speed at appropriate water depths effectively controlled DO concentrations, with a maximum of 6.94 mg/L achieved at a water depth of 25 cm and 20 rpm.
The improvement in DO levels was attributed to increased water circulation, promoting oxygen diffusion. Additionally, higher rotation speeds reduced dead zones from 21.05% to 9.16%, thereby enhancing the overall growth environment for microalgae.
While the study demonstrated the effectiveness of aquavoltaic systems in optimizing microalgae production, it also highlighted the importance of careful parameter management. Lower rotation speeds and deeper water depths could lead to a decrease in DO and pH levels, potentially hindering microalgae growth. Moreover, the economic analysis revealed a slight increase in spirulina production costs with the non-aquavoltaic system due to reliance on non-solar electricity.
Economic analysis
The economic analysis of the study revealed that the aquavoltaic system is more cost-effective than traditional non-aquavoltaic systems. It was found that the cost of spirulina produced with the aquavoltaic system was $0.4975 USD/g at a 5% interest rate and $0.331 USD/g at a 10% interest rate in the fifth year, compared to $0.5445 USD/g with the non-aquavoltaic system.
Implications for the microalgae farming industry
The study’s findings have significant implications for the aquaculture industry, highlighting the potential of aquavoltaic energy to increase microalgae production and reduce production costs.
While aquavoltaic energy offers significant potential, challenges remain, including:
- Initial investment costs: The initial investment to install aquavoltaic systems can be high.
- Technological advancements: Further research and development are needed to optimize system design and improve efficiency.
- Policy support: Government policies and incentives can play a crucial role in promoting the adoption of aquavoltaic energy.
Conclusion
The research highlights the potential of aquavoltaic systems to enhance microalgae production and reduce associated costs. By optimizing key parameters, such as paddlewheel rotation speed and water depth, it is possible to create favorable conditions for microalgae growth while also generating renewable energy.
The researchers conclude that further optimization between energy production and environmental sustainability is needed to fully capitalize on the benefits of aquavoltaic energy. Future studies should explore the long-term impacts of aquavoltaic systems on microalgae productivity, environmental sustainability, and economic viability.
Contact
Shiva Gorjian
Fraunhofer Institute for Solar Energy Systems ISE,
Heidenhofstraße 2, 79110, Freiburg im Breisgau, Germany.
Email: shiva.gorjian@ise-extern.fraunhofer.de, Gorjian@modares.ac.ir
Reference (open access)
Hamedani, H. P., Gorjian, S., Ghobadian, B., & Mokhtarzadeh, H. (2024). Development and experimental performance evaluation of a small-scale aquavoltaic system for microalgae production. Results in Engineering, 24, 102919. https://doi.org/10.1016/j.rineng.2024.102919